Emerging PV Technologies
Solar cell efficiency is the percent of sunlight, measured in photons, that a solar cell absorbs that is converted into usable electricity. A 100% efficient solar cell, for example, would convert 100% of the photons it absorbs into electricity, while a 0% efficient cell would generate no electricity. In 2021, most Silicon solar cells, the standard for home and utility-scale use, have an efficiency between 20-22%.
Scientists, however, have been able to increase the efficiency of PV technology by developing new and innovative solar cells. Improvements to efficiency have so far proved marginal, but are a positive sign for the developing solar field, which hopes to increase the electrical output and overall viability of solar panels.
Vocab
External Load
-
A circuit that makes use of a solar cell's electric current (flow of electrons) for our use as electricity
Redox Potential
-
The likelihood of a material to accept electrons.
Absorption Spectrum
-
The spectrum of light that a material absorbs.
-
A photon's location on the spectrum is determined by its wavelength.
Metal Oxide Semiconductor
-
A semiconductor formed by a metallic element and oxygen.
Dye Sensitized Solar Cells
Dye Sensitized Solar Cells, or DSSCs, are a developing type of solar cell that utilize organic dye to boost electrical efficiency. As an organic solar cell, the DSSC has a fundamentally different makeup than traditional Silicon or Gallium Arsenide based solar cells, and is consequently believed to have a high potential.
DSSC Layers and How They Function
At the most basic level, the layers of a DSSC, in conjunction with an external load, function as segments of a loop. Each layer transports electrons from the previous layer to the next, with the top layer sending electrons to the load and the bottom of the cell receiving electrons from the load. As a result, DSSCs function in a cyclical manner.
The most important layer of a DSSC is the photosensitizer, or organic dye, that absorbs photons. Just like in the n-type side of a Silicon solar cell, the light captured in the dye excites electrons, causing them to break free of their atoms. These freed electrons then begin their journey to the top of the cell, where they are transported to the external load for our use. DSSCs are believed to have high potential because a photosensitizer can absorb more light than the n-type layer of a Si solar cell can, leading to an increase in excited electrons and electricity generation.
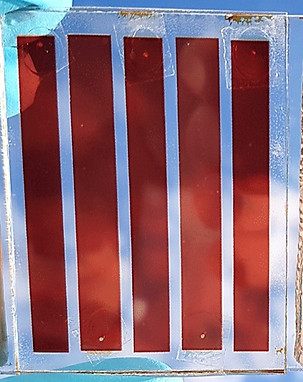
Dye Sensitized Solar Cells.
Image: SyMMES
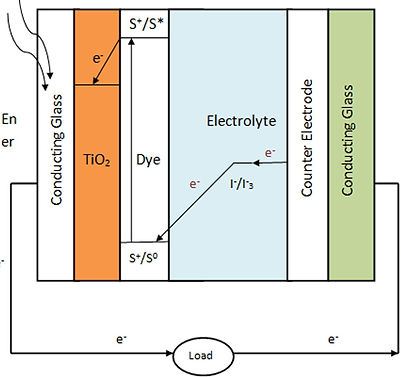
The cross section of a standard DSSC.
Image: Science Direct
Two important factors that determine the effectiveness of an organic dye are its redox potential and absorption spectrum. The higher the redox potential of a dye, the more electrons it will accept from the electrolyte underneath. This allows the dye to “regenerate” faster, meaning that its electron supply is quickly being replenished. With a constant supply of electrons to excite and send to the external load, a dye with a high redox potential will be more efficient than one with a lower redox potential. A photosensitizer with a large absorption spectrum will also heighten the efficiency of a DSSC, as it will absorb a wide range of the electromagnetic spectrum and consequently increase the number of photons available to excite electrons.
The rest of a DSSC is more straightforward. Directly underneath the organic dye is an electrolyte, or a substance that releases ions (charged particles) when dissolved in water. The positively charged ions, known as cations, in the electrolyte accept electrons from the counter electrode beneath and pass them off to the photozensitizer to replenish its supply.
Directly above the organic dye is a metal oxide semiconductor that captures freed electrons and sends them to the top layer of the cell. ZnO (Zinc oxide), TiO2 (Titanium dioxide), Nb2O5 (Niobium pentoxide) and SnO2 (Tin(IV) dioxide) are the most commonly used semiconductors in a DSSC - TiO2 in particular is often viewed as an ideal semiconductor in DSSCs because it is cheap and abundant, nontoxic, and efficient.
Finally, the top and bottom layers of a DSSC are made of a transparent conductive oxide (TCO) glass film. Both layers must be highly conductive, as they facilitate the flow of excited electrons to and from the external load of a cell. The layers are transparent as well, as photons pass through them to reach the photosensitizer beneath. In order to maximize the number of photons that reach the inner layers of a DSSC and boost overall efficiency, the TCO glass, usually ITO (indium tin oxide) or FTO (fluorine tin oxide), has a transparency of over 80%. While ITO has been used by the solar industry for decades and is seen as a reliable material, FTO is cheaper and has been proven to create a more efficient solar cell.
Quantum Dot Solar Cells
The quantum dot solar cell, or QDSC, is a relatively new and increasingly exciting development in the field of photovoltaics. Learning about QDSCs might seem daunting at first because of the word “quantum,” which undoubtedly makes everything sound 100x more interesting and sophisticated. In reality, “quantum” simply indicates that something is incredibly small. A quantum dot solar cell, or a solar cell containing quantum dots, is therefore easily understood as a cell with super small dots on it. These quantum dots are spheres ranging from around 2-10 nanometers in diameter, and are made out of doped semiconductor material the same way that traditional Silicon solar cells are. This material allows quantum dots to absorb photons and free electrons from their atoms.

Quantum dots are applied to a substrate, which in this case is a glass plate, in a few ways: they can be sprayed on, printed on, or, most popularly, spin coated. The main issue with applying quantum dots to a solar cell is that they are difficult to spread out evenly. To do this is both time-consuming and expensive - so expensive, in fact, that it's the most costly part of the manufacturing process of QDSCs.
Once applied, quantum dots replace the function of the n-type side of a Si solar cell by absorbing photons and freeing electrons. Quantum dots absorb photons far more efficiently than traditional Si semiconductors, however, as they commonly free multiple electrons per photon. This phenomenon is called “multiple exciton generation” (MEG for short). After researching MEG, the National Renewable Energy Laboratory, or NREL, proved in 2018 that quantum dots can free up to 3 electrons per photon, showing that the potential maximum efficiency of QDSCs is naturally greater than that of conventional Si cells.
A magnified quantum dot. After being struck by sunlight, the dot releases electrons to the right.
Image: QNA

The absorption spectrum of a quantum dot is determined by its size - smaller dots will absorb higher energy photons, while larger dots absorb lower energy photons. This inverse relationship between dot size and absorbed photon energy makes QDSCs extremely versatile, as scientists can apply various amounts of different sized quantum dots to solar cells in order to produce the most efficient cell possible. For example, a wide range of quantum dots can be used to create a cell with a larger absorption spectrum than conventional PV tech, which generally has a more restricted absorption spectrum. On the other hand, scientists could use only small quantum dots to create a cell that almost exclusively absorbs high energy photons.
The cross section of an experimental QDSC.
Image: J. Phys. Chem. Lett.
As a relatively new development in the solar field, QDSCs have struggled to reach an efficiency viable for use. In 2013 NREL made breakthroughs in the low-cost manufacturing process of QDSCs, but was unable to advance their efficiency beyond 4.4%. After years of steady improvement, however, in 2020 scientists at the Nanomaterials Center of Australia’s University of Queensland were able to construct a QDSC with a 16.6% efficiency. The efficiency of these cells will undoubtedly continue to improve as an increasing amount of research is conducted; according to NREL, QDSCs have the potential to reach a stunning 66% efficiency, more than doubling the projected 31% max efficiency of conventional solar cells.
References
Aslam, Asad, et al. “Dye-Sensitized Solar Cells (DSSCs) as a Potential Photovoltaic Technology for the Self-Powered Internet of Things (IoTs) Applications.” Solar Energy, vol. 207, 1 Sept. 2020, pp. 874–892., doi:https://doi.org/10.1016/j.solener.2020.07.029.
​
Bellini, Emiliano. “Semi-Transparent Dye-Sensitized Solar Module with 8.7% Efficiency.” Pv Magazine International, 11 Dec. 2020, www.pv-magazine.com/2020/12/11/semi-transparent-dye-sensitized-solar-module-with-8-7-efficiency/.
​
Calderone, Len. “Quantum Dot Solar Cells Are Coming.” AltEnergyMag, 22 May 2018, 8:52 AM, www.altenergymag.com/article/2018/05/quantum-dot-solar-cells-are-coming/28547.
​
Honsberg, Christiana, and Stuart Bowden. “Solar Cell Structure.” PVEducation, www.pveducation.org/pvcdrom/solar-cell-operation/solar-cell-structure.
​
Hutchins, Mark. “A Quantum Dot Solar Cell with 16.6% Efficiency.” Pv Magazine International, 19 Feb. 2020, www.pv-magazine.com/2020/02/19/a-quantum-dot-solar-cell-with-16-6-efficiency/.
​
Jacoby, Mitch. “A Powerful Dot Of Solar Energy.” C&EN, 23 Sept. 2013, cen.acs.org/articles/91/i38/Powerful-Dot-Solar-Energy.html.
​
Nguyen, T.C., Can, T.T.T. & Choi, WS. Optimization of Quantum Dot Thin Films using Electrohydrodynamic Jet Spraying for Solution-Processed Quantum Dot Light-Emitting Diodes. Sci Rep 9, 13885 (2019). https://doi.org/10.1038/s41598-019-50181-5
​
“PV Cells 101, Part 2: Solar Photovoltaic Cell Research Directions.” Energy.gov, US Department of Energy, 3 Dec. 2019, www.energy.gov/eere/solar/articles/pv-cells-101-part-2-solar-photovoltaic-cell-research-directions.
​
“Quantum Dots Promise to Significantly Boost Solar Cell Efficiencies.” NREL, National Renewable Energy Laboratory, Aug. 2013, www.nrel.gov/docs/fy13osti/59015.pdf.
​
“Transparent Conductive Oxide (TCO) Substrates.” MSE Supplies LLC, www.msesupplies.com/collections/photovoltaics-solar-cells/fluorine-tin-oxide-fto.
​
“What Are Quantum Dots?” QNA Technology, qnatechnology.com/technology/what-are-quantum-dots/?lang=en.